Educational Appendix
Tumor Microenvironment
A hallmark of cancer is the reprogramming cellular energy metabolism to more effectively support neoplastic proliferation[1]. Cells convert glucose to pyruvate via glycolysis in the cytoplasm and then use pyruvate in oxygen-consuming mitochondria to produce carbon dioxide and ATP[2]. Under anaerobic conditions, pyruvate is primarily converted to lactic acid yielding only 6.25% of the ATP that complete metabolism of glucose would provide through both glycolysis (net 2 ATP) and mitochondrial oxidative phosphorylation (net 30 ATP). In the early 1900's Professor Otto Warburg first reported that cancer cells limit their energy metabolism largely to glycolysis, even in the presence of oxygen[3, 4]. This unique metabolic characteristic is one of the key components leading to a differentiated microenvironment around the tumor cell.[23]
Possible explanations
The Warburg effect is the observation of a high rate of glycolysis (generally associated with anaerobic metabolism) even in the presence of oxygen. Glycolysis provides most of the building blocks required for cell proliferation.[5] Evidence attributes some of the high aerobic glycolytic rates to an overexpressed form of mitochondrially-bound hexokinase.[6] For example, in some kidney cancers, this effect could be due to the presence of mutations in the von Hippel-Lindau tumor suppressor gene upregulating glycolytic enzymes, including the M2 splice isoform of pyruvate kinase (M2-PK).[7] M2-PK is produced in all rapidly dividing cells, and is responsible for enabling cancer cells to consume glucose at an accelerated rate. Upon forcing tumor cells to switch to pyruvate kinase's alternative form by inhibiting the production of tumor M2-PK, their growth was curbed. This enzyme form is not usually found in healthy tissue, though it is apparently necessary when cells need to multiply quickly, e.g. in healing wounds or hematopoiesis.[8][9] Several studies have shown a reduced level of metabolism via oxidative phosphorylation in these cancer cells, which may assist the tumor cells in avoiding apoptosis or programmed cell death.
With an increase in glycolysis and concomitant reduction in oxidative phosphorylation, lactic acid levels increase, so it is not surprising that the tumor microenvironment is frequently characterized by an acidic external pH (pHe).[10]. To maintain an intracellular pH (pHi) that is slightly alkaline (~pH 7.4), tumor cells upregulate several proton extrusion mechanisms such as the Na+/H+ Exchanger (NHE), HCO3− Transporter, Carbonic Anhydrase IX, Vacuolar-ATPase, and the H+/K+ ATPase[11, 12]. Excess protons are excreted into the extracellular matrix, causing the pHe of the tumor microenvironment to become acidic. In certain tumor types such as human MCF-7 mammary carcinoma, the pHe has been measured to be as low as pH 6.44[12]. Chronic exposure to acidic pHe has been reported to promote invasiveness and metastatic behavior in several tumor types[13, 14]. A lower tumor pHe can also contribute to resistance to chemotherapies that act as weak bases, or enhance the efficacy of chemotherapies that act as weak acids[15, 16, 17].In addition low pH is associated with reduced efficacy of immune cells in the tumor microenvironment.
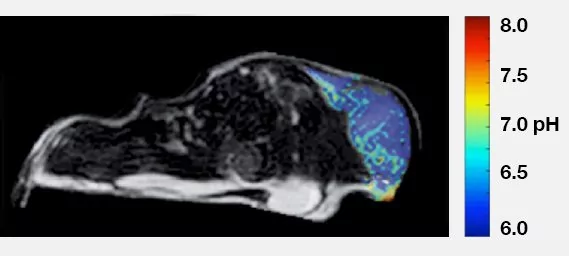
pH of MCF-7 Mouse Xenograft
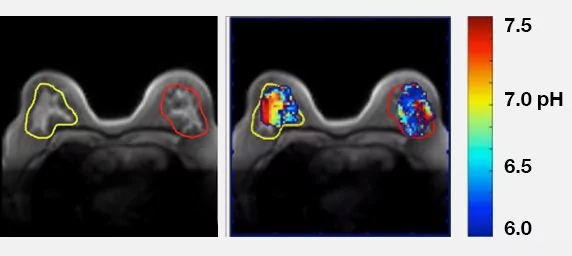
pH of Human Breast Cancer
CREDIT: Courtesy of Professor Marty Pagel, Director of Contrast Agent
Molecular Engineering Lab (CAMEL), University of Arizona Cancer Center
PaCS Mechanism
BioAtla scientists have recently published a paper in PNAS[22] describing a novel mechanism using physiological chemicals as Protein-activated Chemical Switches, or PaCS, for generating CAB antibodies. CAB generation utilizing the PaCS mechanism is applicable to a wide array of antibody formats and antigens, including naked antibodies, ADCs and bispecifics. Existing antibodies can be engineered readily to be made sensitive to PaCS and the tumor selective activity can be optimized for each antigen's varying expression level and tissue distribution.
The ability to engineer CAB antibodies with conditional activity in the Tumor Microenvironment (TME) using the PaCS generation process allows us to target the cell-surface proteins that are relevant cancer targets, but yet exist on normal tissues, where antibody binding outside the TME would cause significant toxicity. The CAB/PaCS system can improve the therapeutic index (TI) for monotherapies and combination therapies, including combining antibodies with checkpoint inhibitors and immune stimulators, which are often not tractable due to systemic toxicity resulting from normal tissue expression.
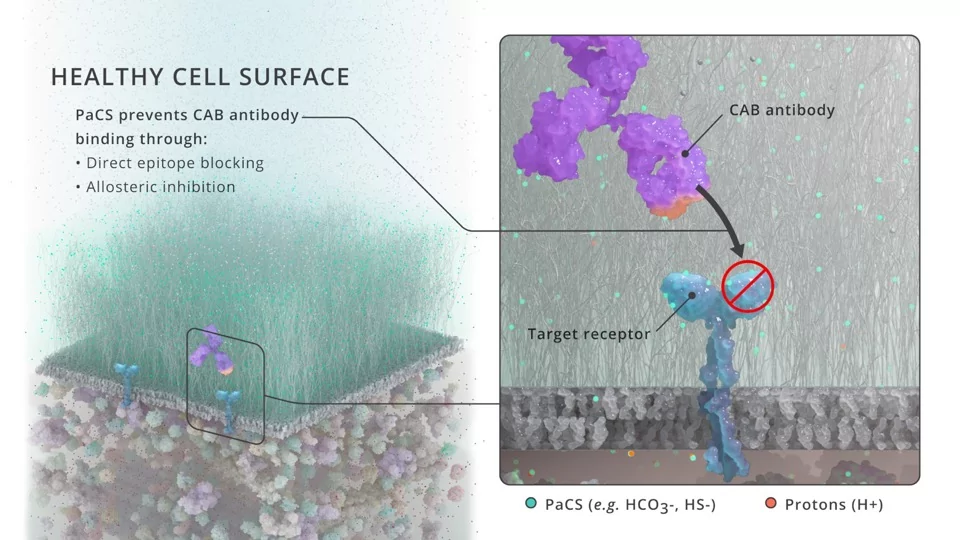
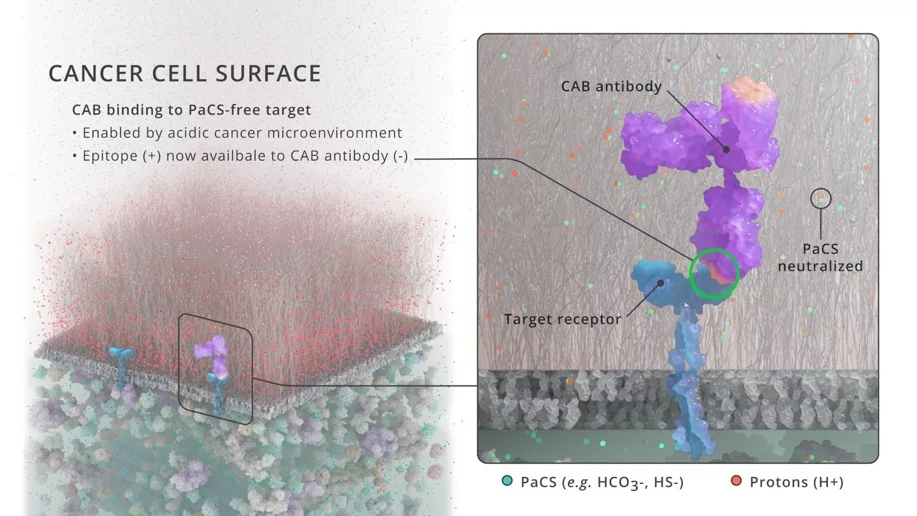
Imaging tumor pH
Professor Marty Pagel and colleagues at the University of Arizona have developed methods to non-invasively measure the extracellular pH (pHe) of the tumor microenvironment in vivo using a magnetic resonance imaging (MRI) method known as chemical exchange saturation transfer (CEST)[4, 18]. To detect a CEST MRI contrast agent, radio frequency saturation is applied at a specific MR frequency of an exchangeable proton on the agent, which reduces the detectable magnetization from this proton. Rapid chemical exchange of this saturated proton with a proton on a near-by water molecule causes the reduced detectable magnetization to be transferred to the water signal. A standard MRI acquisition sequence can then be used to measure the amplitude of the water signal throughout the image. A CEST spectrum is obtained by iterating the saturation frequency and recording the normalized water signal amplitude. Because the average chemical exchange rates of some labile protons are pH-dependent, and because CEST effects from two labile protons can be selectively detected, a ratio of two CEST effects from the same agent can be used to measure pH in a manner that is independent of concentration, magnetic resonance properties of the endogenous tissue, and incomplete saturation.
Professor Pagel and colleagues have termed the use of in vivo CEST MRI for measuring tumor acidosis "acidoCEST MRI"[19, 20]. Their image acquisition method uses an in vivo CEST-FISP MRI protocol that rapidly detect in vivo CEST effects, which is critical for tracking exogenous contrast agents within in vivo tissues. Our image analysis method uses Lorentzian line shape fittings that improve the precision of in vivo CEST measurements. They detect the CEST signals of iopamidol (Isovue™, Bracco Diagnostics, Inc.) that is administered to a mouse tumor model, to create a method that can noninvasively measure tumor pHe. Together, these technological developments result in a measure precision of 0.07 pH units and an accuracy of 0.034 pH units.
To demonstrate the utility of acidoCEST MRI, they have for example treated a Raji xenograft tumor model of lymphoma with MIBG which reduces mitochondrial activity[21]. They performed acidoCEST MRI before treatment and 4 hours after treatment with MIBG and measured a drastic decrease in average tumor pHe, indicating that this mitochondrial poison caused metabolism of glucose to shift towards anaerobic glycolysis and presumably produce substantial amounts of lactic acid. Thus, acidoCEST MRI may be very useful in better understanding cancer cell metabolism and how to exploit it in cancer treatment.
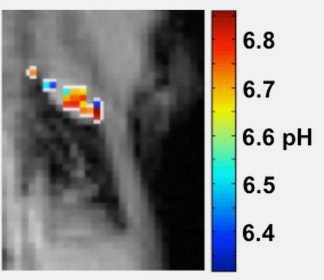
Human Lung Cancer
CREDIT: Courtesy of Professor Marty Pagel, Director of Contrast Agent Molecular Engineering Lab (CAMEL), University of Arizona Cancer Center
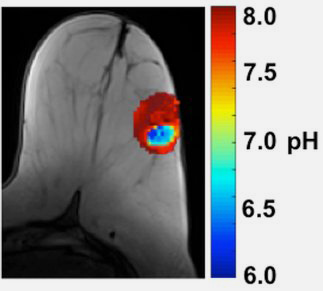
Human Breast Cancer
CREDIT: Courtesy of Professor Marty Pagel, Director of Contrast Agent Molecular Engineering Lab (CAMEL), University of Arizona Cancer Center
References
- Hanahan and Weinberg RA (2011) Hallmarks of cancer: the next generation. Cell 144(5): 646-674.
- Beddek AJ et al. (2008) Profiling the metabolic proteome of bovine mammary tissue. Proteomics 8(7): 1502-1515.
- Warburg O (1956) On the origin of cancer cells. Science 123(3191): 309-314.
- Chen LQ and Pagel MD (2015) Evaluating pH in the extracellular tumor microenvironment using CEST MRI and other imaging methods. Advances in Radiology 2015, article ID 206405.
- Lopez-Lazaro M (2008) The Warburg effect: why and how do cancer cells activate glycolysis in the presence of oxygen? Anticancer Agents Med. Chem. 8(3): 305-312.
- Bustamante E and Pedersen PL (1977) High aerobic glycolysis of rat hepatoma cells in culture: role of mitochondrial hexokinase. Proc. Natl. Acad. Sci. U.S.A. 74(9): 3735-3739.
- Unwin RD et al. (2003) Proteomic changes in renal cancer and co-ordinate demonstration of both the glycolytic and mitochondrial aspects of the Warburg effect. Proteomics 3(8): 1620-1632.
- Christofk HR et al. (2008) The M2 splice isoform of pyruvate kinase is important for cancer metabolism and tumour growth. Nature 452(7184): 230-233.
- Pedersen PL (2007) Warburg, me and Hexokinase 2: Multiple discoveries of key molecular events underlying one of cancers' most common phenotypes, the "Warburg Effect", i.e., elevated glycolysis in the presence of oxygen. J. Bioenerg. Biomembr. 39(3): 211-222.
- Griffiths JR (1991) Are cancer cells acidic? Br J Cancer 64:425-427.
- Gillies RJ et al. (2004) pH imaging. IEEE Engineering in Medicine and Biology Magazine 23(5): 57-64.
- Gillies RJ et al. (2002) MRI of the tumor microenvironment. Journal of Magnetic Resonance Imaging 16(4): 430-450.
- Martınez-Zaguilan R. et al. (1996) Acidic pH enhances the invasive behavior of human melanoma cells. Clinical and Experimental Metastasis (14)2: 176-186.
- Gillies RJ and Gatenby RA (2007) Hypoxia and adaptive landscapes in the evolution of carcinogenesis. Cancer and Metastasis Reviews 26(2): 311-317.
- Mahoney BP et al. (2003) Tumor acidity, ion trapping and chemotherapeutics. I. Acid pH affects the distribution of chemotherapeutic agents in vitro. Biochem Pharmacol 66: 1207-18.
- Raghunand N et al. (2003) Tumor acidity, ion trapping and chemotherapeutics II. pH-dependent partition coefficients predict importance of ion trapping on pharmacokinetics of weakly basic chemotherapeutic agents. Biochem Pharmacol 66: 1219-29.
- Gerweck LE and Seetharaman K. (1996) Cellular pH gradient in tumor versus normal tissue: potential exploitation for the treatment of cancer. Cancer Res 56: 1194-8.
- Liu G et al. (2012) Imaging in vivo extracellular pH with a single paramagnetic chemical exchange saturation transfer magnetic resonance imaging contrast agent. Molecular Imaging 11(1): 47-57.
- Chen LQ et al. (2013) Evaluations of extracellular pH within in vivo tumors using acidoCEST MRI. Magnetic Resonance in Medicine 72(5): 1408-1417.
- Chen LQ et al. (2015) Evaluations of tumor acidosis within in vivo tumor models using parametric maps generated with AcidoCEST MRI. Mol Imaging Biol 17(4): 488-96.
- Chen LQ et al. (2015) Assessment of Carbonic Anhydrase IX expression and extracellular pH in B-cell lymphoma cell line models. Leukemia & Lymphoma 56(5): 1432-143.
- Chang et al. (2021) Generating tumor-selective conditionally active biologic anti-CTLA4 antibodies via protein-associated chemical switches.
- N. Rohani et al., Acidification of tumor at stromal boundaries drives transcriptome alterations associated with aggressive phenotypes. Cancer Res. 79, 1952-1966 (2019).